Introduction
Over 55 million people have dementia worldwide, and their number is expected to increase to 78 million in 2030. Approximately 139 million in 2050 as the proportion of the older population continues to increase globally. According to the population estimate of the National Statistical Office, Korea has the fastest rate of aging population worldwide, with a projection of 46.5 % of the total population being 65 years of age or older in 2045 (Statistics Korea).
Alzhemier’s disease (AD) is a progressive neurodegenerative disease characterized by the degeneration and loss of neurons and synapses throughout the brain in aging individuals and patients with AD. The memory and cognitive functions of patients with AD progressively decline, with patients developing dementia and dying prematurely (Terry & Davies, 1980). With the increase in the number of older people, the number of patients with AD is expected to double every 17 years. It is estimated to reach 1 million in 2024 and 3 million in 2050 (Arvanitakis et al., 2019). There are numerous ongoing studies on therapeutic candidates for AD; however, we currently lack drugs for the treatment or prevention of AD, partly owing to the insufficient understanding of the underlying pathogenic mechanisms (Cummings et al., 2021; Huang et al., 2020).
Several animal models of AD have been used in pharmaceutical research and drug development. Typical animal models of AD include spontaneously aging animals, transgenic animals, and chemically induced animal models (Drummond & Wisniewski, 2017; Esquerda-Canals et al., 2017). Spontaneous aging and transgenic animal models are widely used in AD studies because their pathology is similar to that of humans. However, these models have certain disadvantages, such as being time-consuming, demanding breeding management, and increased cost (Park et al., 2020; Park et al., 2013). Chemically induced animal models using amyloid beta (Aβ), cholinotoxin, and cholinergic antagonists are relatively economical. However, they have other disadvantages, such as short memory retention, and sometimes require highly professional skills (i.e., intracerebroventricular injection). Therefore, in the drug research and development for AD, several models are used in combination to evaluate the tested drugs according to their pharmacological mechanisms (Gilles & Ertle, 2000; Nakai et al., 2021; Park et al., 2012; Zeiss, 2020).
Among the chemicals that cause AD, AF64A is a choline analog that is internalized only into cholinergic neurons by the high-affinity choline transport system, causing alterations in the mRNA expression and activity of choline acetyltransferase (ChAT) (Bessho et al., 2008; Fan & Hanin, 1999; Fisher et al., 1982; Yamazaki et al., 1991). Hence, injection of AF64A decreased the release of acetylcholine (ACh), leading to cognitive impairments, including memory and learning deficits, a salient characteristic of AD (Abe et al., 1993; Bessho et al., 2008; Yamazaki et al., 1991). AF64A is a cholinergic toxin; therefore, it is mainly used to develop drugs related to the cholinergic pathway but not to amyloid beta, the causative agent of AD.
In this study, we analyzed the changes in the levels of inflammatory mediators and proteins related to the cholinergic and amyloidogenic pathways in AF64A-induced AD. In addition, we performed memory tests in AF64A-induced AD rats four weeks after model establishment to examine the possibility of using this animal model for efficacy testing in drug development and provide basic information on animal models of AD.
Materials and Methods
AF64A Preparation
AF64A was freshly prepared from acetyl-AF64 using the method described by Nakahara et al. (1988). Briefly, 16 mg acetyl-AF64 (Sigma-Aldrich, St. Louis, MO, USA) was rapidly dissolved in 6.4 mL distilled water under constant stirring. The pH was then adjusted and maintained between 10.5 and 11.0 by adding NaOH solution, as required. The reaction was allowed to proceed for approximately 25 min until no rapid pH drop was observed. After termination of the reaction, HCl solution was added to reduce the pH once to 5.5–7.0, and the final pH was adjusted to 7.4 by NaOH solution. Distilled water was added to bring the final concentration of AF64A to 10.0 mM. This stock solution was diluted with PBS to 50 μM for cell culture and 5 μM for animal experiments.
Acetylcholinesterase (AChE) Activity in the Brain
Nine-week-old Sprague-Dawley rat brains were quickly collected after transcardial perfusion with cold saline under anesthesia and homogenized by adding 9 times PBS supplemented with a protease inhibitor cocktail (Sigma-Aldrich). AChE activity was assayed at 25℃ using the method described by Ellman et al. (1961) with slight modifications. Briefly, the assay mixture (1.0 mL PBS) contained 33 μL of 1.5 mM acetylcholine iodide and 33 μL of 1.5 mM 5.5′-dithiobis-(2-nitrobenzoic acid). Subsequently, 100 μL AF64A (1–1,000 μM) and brain homogenate (50 μL) were added to the assay mixture, and the change in absorbance at 412 nm over 5 min was measured using a spectrophotometer (SpectraMax M2, Molecular Devices, San Jose, CA, USA). The relative enzymatic activity (inhibited by AF64A) was calculated based on the change in the absorbance (full activity: 100 %) of the assay mixture containing 100 μL PBS without AF64A.
Cell Culture
To evaluate the effect of AF64A on human neural stem cells (hNSCs), cells were cultured in Dulbecco’s modified Eagle’s medium (DMEM; Biowest, Nuaillé, Cholet, France) containing antibiotics (100 IU/mL penicillin and 100 μg/mL streptomycin) and 10 % heat-inactivated fetal bovine serum (Biowest) (Kim et al., 2008). Briefly, hNSCs were incubated with 50 μM AF64A for 24 h at 37℃ in a 5 % CO2/95 % air atmosphere. In all experiments, cells were grown until they reached 90 % confluence and subjected to a maximum of 20 passages.
Animals and Treatment
Five-week-old male Sprague-Dawley rats were purchased from Daehan Biolink (Eumseong, Korea). Rats were housed in an environmentally controlled room under constant temperature (23 ± 3℃), relative humidity (50 ± 10 %), and a 12 h light/dark cycle. Rats were fed a standard rodent diet and purified water ad libitum. One week later, rats were anesthetized with isoflurane, positioned in a stereotaxic frame, and administered AF64A to induce cognitive deficits. After incision of the skin and drilling a hole, a freshly-prepared AF64A solution (5 μM/5 μL/rat) was infused into the right ventricle at the following stereotaxic coordinates from bregma: posterior 0.8 mm, lateral 1.5 mm, and ventral 4.0 mm, at a flow rate of 0.5 μL/min. All animal experiments were performed in accordance with the standard operating procedures of the Laboratory Animal Center, Chungbuk National University (CBNU), Korea. The protocol was approved by the Institutional Animal Care and Use Committee of CBNU (#CBNUA-1527-21-02).
Quantitative Real-time PCR Analysis
Total RNA was isolated from hNSCs and brain tissues using TRIzol Reagent (Invitrogen, Carlsbad, CA, USA) according to the manufacturer’s instructions. Quantitative real-time PCR was performed as previously described (Yon et al., 2018). Glyceraldehyde 3-phosphate dehydrogenase (GAPDH) was used as an internal standard to normalize the expression of target transcripts. The primers used to amplify nuclear factor-kappa B (NF-κB), tumor necrosis factor-α (TNF-α), interleukin-6 (IL-6), inducible nitric oxide synthase (iNOS), and cyclooxygenase-2 (COX2) are provided in Table 1. Triplicate data from three independent assays were analyzed using the comparative Ct method (Yon et al., 2018).
Western Blot Analysis
hNSCs and brain tissues were homogenized in 10 volumes of RIPA buffer (Thermo Fisher Scientific, Waltham, MA, USA) containing protease (Sigma-Aldrich) and phosphatase (Sigma-Aldrich) inhibitors. Western blotting was performed as previously described (Yang et al., 2013). Membranes were immunoblotted using respective primary antibodies, followed by incubation with horseradish peroxidase-conjugated anti-rabbit and anti-mouse secondary antibodies (Jackson ImmunoResearch Laboratories, Inc., West Grove, PA, USA). The antibodies used in this study are listed in Table 2. Band densities were measured using the ImageJ software (NIH, Bethesda, MD, USA) and normalized to that of actin.
Passive Avoidance Test
Rats were subjected to a step-through shuttle box (ENV-010MD; Med Associates, St. Albans, VT, USA) once a day for six days starting four weeks after the AF64A injection to evaluate memory acquisition. The shuttle box apparatus consisted of 2 compartments equipped with a lamp and steel-grid floor for electric shock. In the trials, rats were subjected to electric shock (0.4 mA for 2 s) when they entered the dark compartment from the lightroom through a guillotine door. The latency time of stay was recorded when the light in the light room was turned on, and the end-point denoting full acquisition of memory was set to 180s.
Morris Water Maze Test
The spatial memory of rats was evaluated using a Morris water maze system (Smart v2.5; Panlab Technology, Barcelona, Spain) connected to a CCTV (Samsung, Changwon, Korea) with the same daily schedule as that used for passive avoidance performance (2 h later). A circular water bath (180 cm in diameter) was used for the water-maze trials, with water filled to a depth of 27 cm maintained at a temperature of 22 ± 2℃. The water bath formed 4 quadrants and had a hidden escape platform (10 cm in diameter and 25 cm in height) located in the center of 1 quadrant, 2 cm below the surface of the water. The platform was hidden by squid ink in the water, and rats were trained to find the platform based on several cues placed external to the maze. The positions of these cues were kept constant throughout the experiments. The endpoint was set at 180 s if the animal failed to find the platform. The escape latency of rats, that is, the time required to escape onto the platform during the trials, was recorded by tracking with the CCTV monitor.
Statistical Analysis
For statistical comparisons between groups, normality and homogeneity of variance were confirmed, and Student’s t-tests were performed. All analyses were conducted using the Statistical Package for Social Sciences for Windows software (version 12.0; SPSS Inc., Chicago, IL, USA). Statistical significance was set at p<0.0.05. All data are expressed as the mean ± SD.
Results
AChE Activity Using Brain Tissue
To measure AChE activity, we added various concentrations (0–1000 μM) of AF64A to the assay mixture and brain homogenate. We observed that lower concentrations (<250 μM) of AF64A did not decrease AChE activity (Fig. 1). In contrast, AChE activity was decreased when the concentration of AF64A was > 250 μM. Notably, we observed that AChE activity was significantly decreased at 500 and 1,000 μM AF64A compared with that in vehicle control.
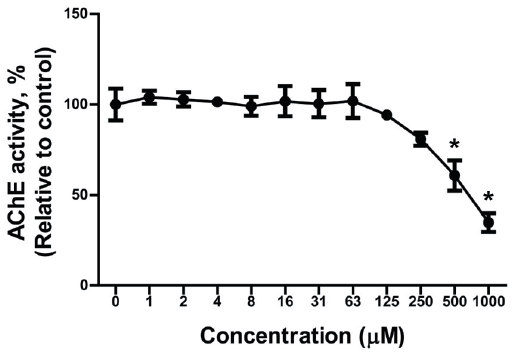
Fig. 1. Acetylcholinesterase (AChE) activity using brain tissue. For this assay, 100 μL AF64A (0–1,000 μM) was added to the assay mixture (1.0 mL PBS) containing 33 μL of 1.5 mM acetylcholine iodide, 33 μL of 1.5 mM 5.5′-dithiobis-(2-nitrobenzoic acid), and 50 μL brain homogenate. Data are expressed as the mean ± SD. All samples were analyzed in triplicate. *indicates significantly different from vehicle (p<0. 0.05).
Inflammatory Mediators in hNSCs and Brain Tissue after AF64A-induced Damage
To analyze the inflammatory response induced by AF64A, we synthesized cDNA from hNSCs and brain tissues four weeks after injection of AF64A, followed by real-time PCR (Figs. 2 and 3). We observed that treatment of hNSCs (50 μM) and brain tissues (5 μM) with AF64A significantly upregulated the expression of NF-κB. Meanwhile, we also observed that AF64A substantially increased the expression of TNF-α and IL-6. Consistent with the upregulation in the levels of NF-κB and inflammatory cytokines, the gene expression levels of inflammatory enzymes, iNOS and COX2, were increased following AF64A exposure.
Cholinergic and Amyloidogenic Pathways in hNSCs and Brain Tissues after AF64A-induced Damage
To analyze the effect of AF64A in the cholinergic system, we compared the protein expression hNSCs and brain tissues four weeks after injection of AF64A related to acetylcholine synthesis by western blotting (Fig. 4). We observed that the expression of choline transporter 1 (ChT1), choline acetyltransferase (ChAT), and vesicular acetylcholine transporter (VAChT) was significantly decreased in the AF64A-treated group compared with that in the normal group.
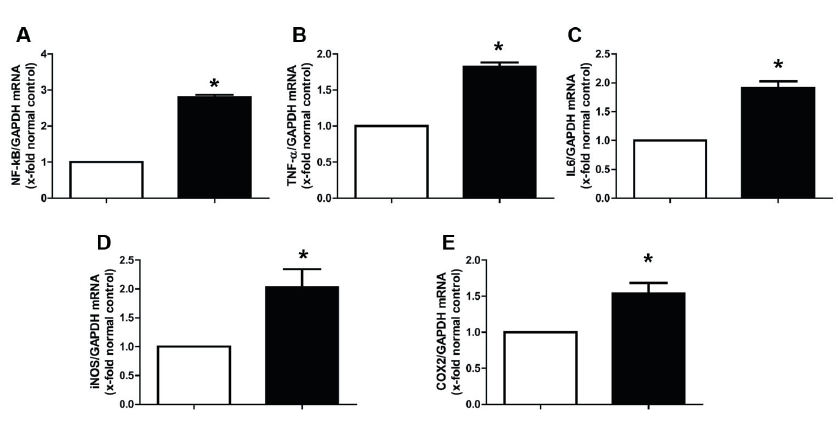
Fig. 2. mRNA expression of inflammatory mediators in human neural stem cells (hNSCs) treated with AF64A. mRNA expression of nuclear factor kappa B (NF-κB, A), tumor necrosis factor-α (TNF-α, B), interleukin-6 (IL-6, C), inducible nitric oxide synthase (iNOS, D), and cyclooxygenase-2 (COX2, E), normalized to GAPDH. White: normal; black: treatment with AF64A. Data are expressed as the mean ± SD. All samples were analyzed in triplicate. *indicates significantly different from control (p<0. 0.05).
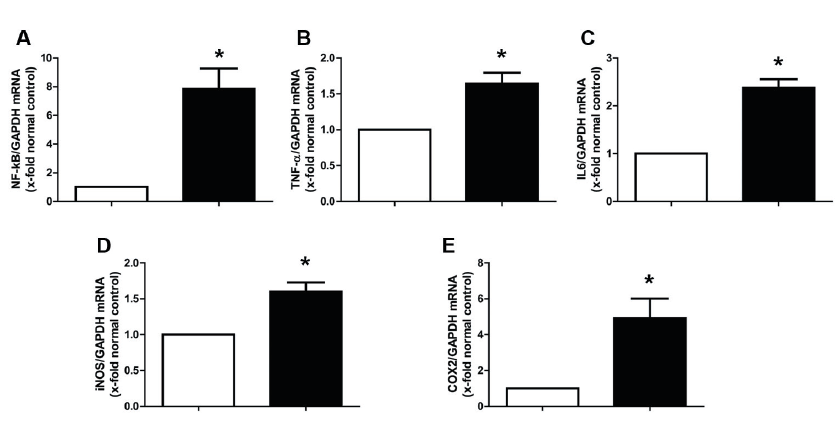
Fig. 3. mRNA expression of inflammatory mediators in brain tissues of animals treated with AF64A. mRNA expression of NF-κB (A), TNF-α (B), IL-6 (C), iNOS (D), and COX2 (E), normalized to GAPDH. White: control animal; black: animal treated with AF64A. Data are expressed as the mean ± SD. All samples were analyzed in triplicate. *indicates significantly different from control (p<0. 0.05).
In addition, we observed that the expression of beta-secretase1 (BACE1), which is related to Aβ synthesis, was increased by AF64A in the amyloidogenic pathway (Fig. 4). However, we noticed that the expression of insulin-degrading enzyme (IDE) and neprilysin, which are related to the degradation of Aβ, was decreased by AF64A. Consistent with these changes in the amyloidogenic pathway, we detected that the expression of Aβ was also substantially increased following exposure to AF64A.
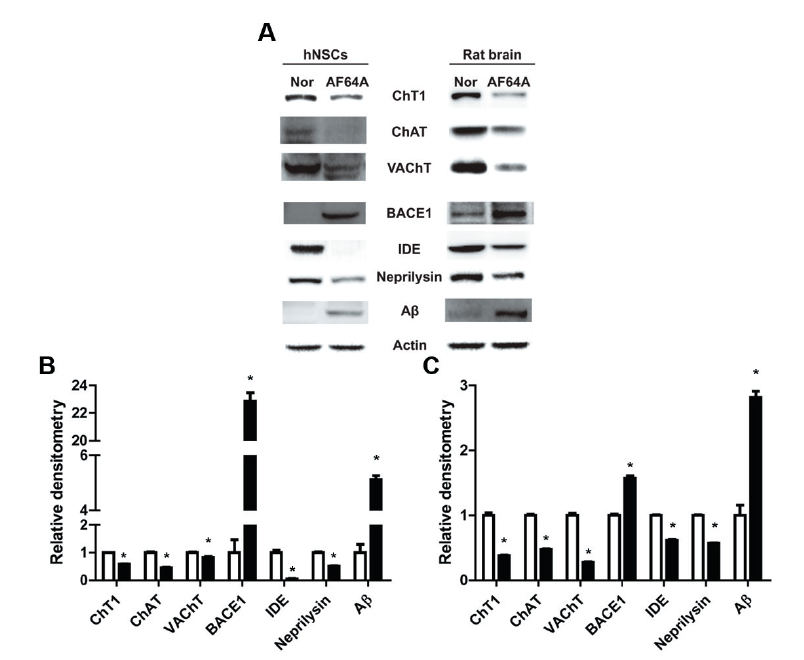
Fig. 4. Expression of protein related to the cholinergic and amyloidogenic pathways in hNSCs and brain tissues of animals treated with AF64A. (A) Representative bands of choline transporter 1 (ChT1), choline acetyltransferase (ChAT), and vesicular acetylcholine transporter (VAChT) proteins, which are related to the cholinergic pathway, and those of beta-secretase1 (BACE1), insulin-degrading enzyme (IDE), and neprilysin proteins, which are related to the amyloidogenic pathway. (B and C) Band densities were normalized to that of actin. White: control animal; black: animal treated with AF64A. Data are expressed as the mean ± SD. All samples were analyzed in triplicate. *indicates significantly different from control (p<0.0.05).
AF64A-induced Memory Deficit
To confirm the AF64A-induced memory deficit, we subjected rats to passive avoidance (Fig. 5A) and Morris water maze (Fig. 5B) tests four weeks after injection of AF64A. In the passive avoidance test that evaluates the conditioned memory in the hippocampus and memory associated with the limbic system, we observed that the normal group learned to avoid the electric shock in the dark room and gradually retained this memory in the bright room as training progressed. However, despite the electric shock, the group treated with AF64A exhibited a short memory retention time owing to memory loss. In particular, we observed that this difference in memory retention was apparent on day 4 and was significantly enhanced on days 5 and 6.
To confirm the AF64A-induced memory loss, we subjected rats to the Morris water maze test to evaluate their spatial memory. Consistent with the results of the passive avoidance test, we observed that rats in the control group demonstrated considerably reduced time to find the platform. In contrast, rats treated with AF64A demonstrated minimal change in their escape times regardless of the training period. In particular, we observed that these differences between the two groups were apparent on day 4 and were significantly enhanced on days 4 and 6. Similar to the escape time, the distance covered was also increased in the group treated with AF64A compared with that in the normal group (Fig. 5C and D).
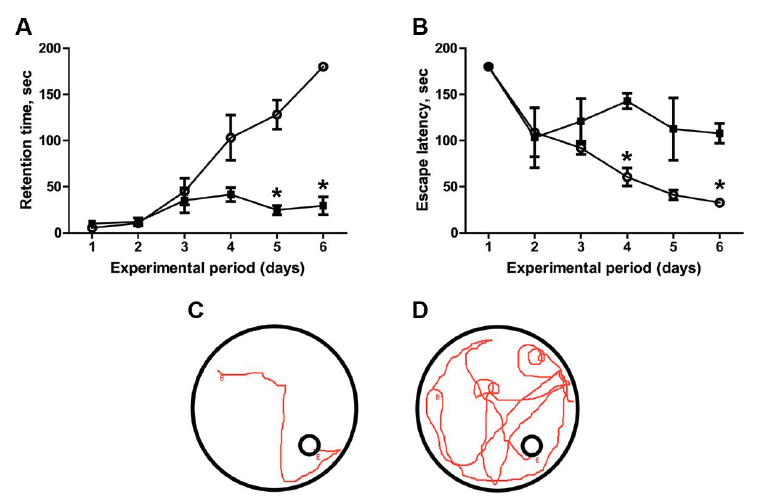
Fig. 5. Expression of protein related to the cholinergic and amyloidogenic pathways in hNSCs and brain tissues of animals treated with AF64A. (A) Representative bands of choline transporter 1 (ChT1), choline acetyltransferase (ChAT), and vesicular acetylcholine transporter (VAChT) proteins, which are related to the cholinergic pathway, and those of beta-secretase1 (BACE1), insulin-degrading enzyme (IDE), and neprilysin proteins, which are related to the amyloidogenic pathway. (B and C) Band densities were normalized to that of actin. White: control animal; black: animal treated with AF64A. Data are expressed as the mean ± SD. All samples were analyzed in triplicate. *indicates significantly different from control (p<0.0.05).
Discussion
In this study, we examined the alterations in the levels of inflammatory mediators, the activity of the cholinergic system and the amyloidogenic pathway, and evaluated the learning and memory abilities of rats with AF64A-induced neurodegeneration.
The cause of AD is unknown; however, two theories are currently accepted. The first considers neuronal death owing to Aβ accumulation a major cause, whereas the second considers the damage to neuronal cells owing to tau protein peroxidation (Ashrafian et al., 2021). Accumulated Aβ destroys cholinergic nerves and causes memory deficits, whereas peroxidation of tau also disrupts synaptic transmission, leading to memory dysfunction (Gao et al., 2018; Pinheiro & Faustino, 2019). Although recently developed drugs are aimed at removing these causative agents, no effective treatments have yet been developed.
Consequently, the drugs currently being developed aim to alleviate symptoms, such as learning and memory dysfunction and cognitive deficits; one such drug is donepezil. In addition, many studies on the potential protective and therapeutic effects of natural products are being conducted in Korea. Several animal models of neurodegeneration induced by scopolamine, LPS, or AF64A, and transgenic animal models are used to develop drugs (Ferreira-Vieira et al., 2016; Gao et al., 2018; Oh et al., 2021).
We observed that in the AChE activity test, there was no significant difference in the AChE activity at a low dose of AF64A compared with that of the vehicle. In contrast, the AChE activity was significantly decreased at a high dose of AF64A. AChE is an enzyme that degrades ACh in synapses (Trang & Khandhar, 2022). This observed decrease in AChE activity led to a temporary increase in the action time of ACh owing to the inhibition of the degradation of ACh. Drugs, such as donepezil and tacrine, which are currently being used as treatments, temporarily increase the concentration of ACh by suppressing AChE activity (Akasofu et al., 2008; Marucci et al., 2021; Shin et al., 2018). In this study, we demonstrated that AF64A decreased AChE activity, consistent with other studies (Leventer et al., 1987). However, in the present study, the decrease in AChE activity appeared only at high concentrations, whereas no significant difference from the control was observed in concentrations used in cell or animal experiments; therefore, we considered that it did not have a significant impact on efficacy tests.
Notably, Aβ accumulation increases the inflammatory response and damage to neuronal cells, leading to cell death (Kinney et al., 2018). In this study, AF64A significantly increased the expression of members of inflammatory signaling pathways, such as NF-κB, inflammatory cytokines, such as TNF-α and IL-6, and inflammatory mediators, such as iNOS and COX2, compared with that in the normal group. These changes are common symptoms in all AD animal models (Kitazawa et al., 2005; Zhao et al., 2019). Notably, the AF64A-induced decrease in the levels of acetylcholine is thought to affect the increase in the levels of inflammatory cytokines (Sun et al., 2013).
Many studies have reported that cognitive dysfunction in patients with AD is caused by damage to the cholinergic nerve (Gais & Born, 2004; Rogers & Kesner, 2003). ACh is a neurotransmitter closely related to memory formation, and a decrease in the concentration of ACh has been reported to be associated with learning and memory dysfunctions (Haam & Yakel, 2017; Hasselmo, 2006). Therefore, the expression of proteins related to acetylcholine synthesis is reduced in AD animals; concordantly, they are sometimes used in AD animal modeling (Mannisto et al., 1994). AF64A is the most representative cholinotoxin (Mannisto et al., 1994; Park et al., 2012), and in this study, we confirmed that the expression of ChT1, ChAT, and VAChT, which are related to Ach synthesis, was significantly reduced after AF64A treatment compared with that in the normal group. Therefore, we believe that AF64A can be used to evaluate the efficacy of precursors for ACh synthesis in animal models.
Notably, AF64A also affected the amyloidogenic pathway. As is known, AF64A causes cell death of the cholinergic system, resulting in increased neuroinflammation. Therefore, neuroinflammation induced brain damage, amyloid genesis and memory deficit (Noh et al., 2014). Furthermore, brain inflammation is accompanied by neuronal loss and microglia activation, which induce the release of neurotoxic factors, such as inflammatory cytokines. Increasing levels of inflammatory cytokines have been reported to increase the expression of proteins associated with the generation of Aβ (Alasmari et al., 2018; Sommer et al., 2017). Based on this, several studies have been conducted on animal models after inducing memory deficits by intracerebroventricular injection of LPS (Kitazawa et al., 2005; Zhao et al., 2019). However, the effect of AF64A on the amyloidogenic pathway has rarely been explored. We specifically observed that AF64A increased the expression of Aβ-generating BACE1, whereas it decreased the expression of Aβ-degrading neprilysin and IDE through inflammatory response. This suggested that the AF64A-induced neurodegeneration model can be used in ACh-related research, as well as in Aβ-related research.
In addition, the AF64A-induced neurodegeneration animal model demonstrated advantages in passive avoidance and Morris water-maze tests. Typically, scopolamine- or LPS-induced AD animal models are used in studies investigating the long-term preventive rather than therapeutic effects of drugs owing to memory recovery within a week (Go et al., 2022; Yoon et al., 2022). However, our AF64A-induced neurodegeneration animal model could be used in studies that evaluate therapeutic effects, as we confirmed the stably dysfunctional memory of animals even after four weeks.
Conclusions and Implications
In conclusion, in the AF64A-induced neurodegeneration animal model, AF64A acted as a cholinotoxin to reduce the expression of ChT1, ChAT, and VAChT proteins related to acetylcholine synthesis. In contrast, it increased the expression of NF-κB, TNF-α, IL-6, iNOS, and COX2, thereby increasing the inflammatory response. Consequently, when subjected to behavioral tests, the respective animals exhibited memory deficiency owing to defective expression of proteins related to the amyloidogenic pathway, such as BACE1, neprilysin, and IDE. Therefore, the AF64A-induced neurodegeneration model can be valuable in ACh-related research as well as in Aβ-related research, and could be employed in the evaluation of the therapeutic effect of drugs as memory deficits are maintained in this model for over four weeks.
Authors Information
Yoon, Eun-Jung: Department of Biology Education, Korea National University of Education, Researcher, First Author
Choi, Yunseo: Department of Biology Education, Korea National University of Education, Graduate Student, Co-author
Park, Dongsun: Korea National University of Education, Professor, Corresponding Author